How can astronomers know what a star is made of if we can’t go out and take samples?
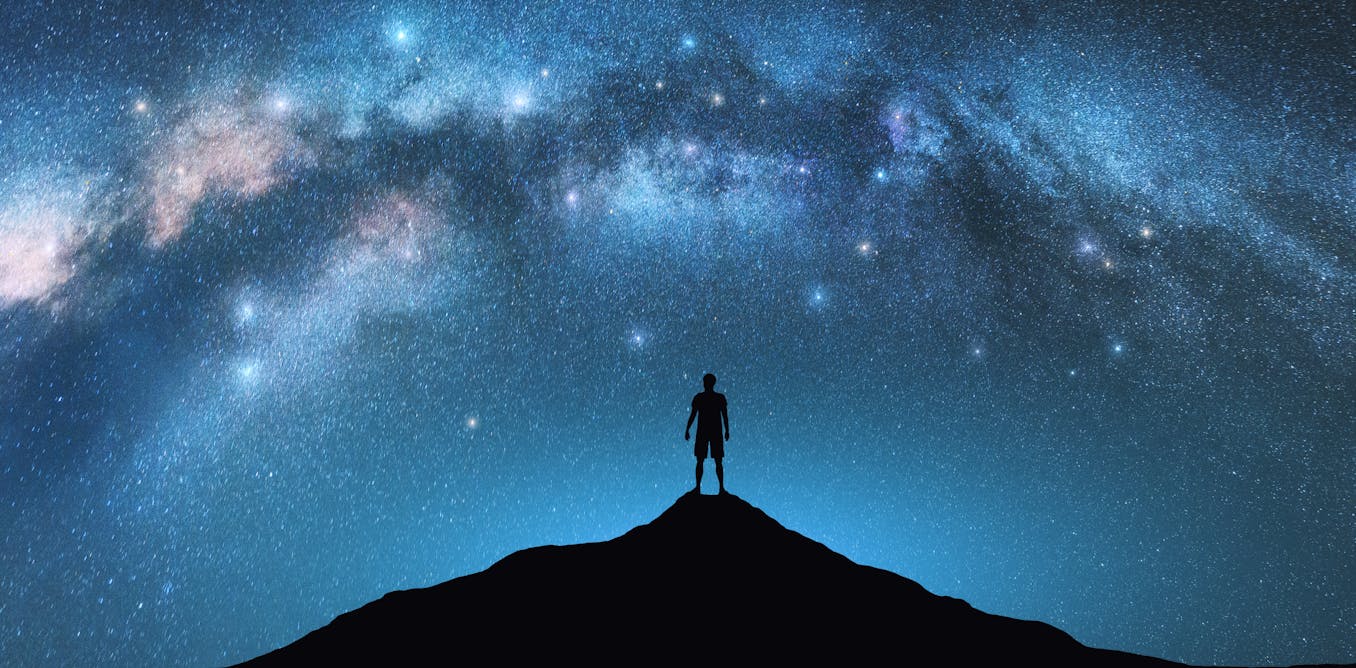
The spice store clerks have incredible gifts. Just by looking at the powder, they can identify it and know whether it is baking soda, baking powder, sodium benzoate, or any other ingredient. On the contrary, some mere mortals cannot distinguish salt from sugar, which leads to sad culinary consequences.
How can we determine the composition of something if we do not have the above-mentioned gift? The answer is simple: you need to experiment. We can appreciate its texture, smell it and, if we dare, even taste it. Moreover, we could include it in various recipes to test its effect on bread, on the cooking temperature of meat, or on its ability to create gastronomic explosions.
The experimental approach described above is not much different from what has been done for thousands of years to study chemical composition. They experimented with all types of materials, many of which were dangerous. Among the pioneers, some suffered burns, poisoning, poisoning, suffocation and even death from radiation. This was hard-won experimental knowledge.
To determine the composition of things, we relied on direct experiments. The possibility of studying distant stars seemed unattainable. In 1830, philosopher Auguste Comte expressed his pessimism by declaring that we would never be able to determine the chemical composition of stars.
Fortunately for everyone, Comte was wrong.
Over the Rainbow
As is often the case in science, help came from a seemingly unrelated area.
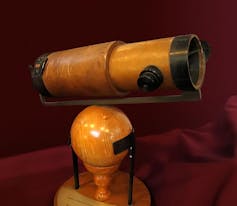
Wikimedia Commons, CC BY
In 1665, Isaac Newton conducted his famous optical experiments. He passed a beam of sunlight through a prism, reproducing the visible spectrum of a rainbow.
Later, in 1800, William Herschel discovered a form of light invisible to the human eye, revealed by its heat. He repeated Newton’s experiment by placing thermometers of different colors. Thus, he discovered that heat was detected beyond the red, where light did not appear to reach. He called this invisible light “caloric rays,” a term immortalized by H. G. Wells in his novel. War of the Worlds.
Herschel’s discovery of what we today call infrared radiation was only the first step. Then came microwaves, radio waves, ultraviolet radiation, x-rays and gamma rays. Our seven-color rainbow expanded to form what we now know as the “electromagnetic spectrum.”
Absorption, emission and continuous spectra
In 1814, Joseph Fraunhofer discovered dark lines in the solar spectrum. It seemed as if the light of these flowers had been absorbed.
On the other hand, Thomas Melville, from 1752, observed something strange when burning salts. Their spectrum was not a continuum of colors, but a series of specific bright bands.
Today we call the “absorption spectrum” the one in which there are dark bands, and the “emission spectrum” formed by the specific bands. A spectrum without dark bands is called a “continuous spectrum.”
The spectrum speaks more than a thousand images
We all know that a picture speaks more than a thousand words, but the amount of information contained in spectra far exceeds this rule. Light has different emission mechanisms.
Thermal radiation is the result of a dynamic change in the speed of charged particles in a body. Warmer objects contain particles with significantly higher average velocities, causing more intense and energetic velocity changes than cooler objects. They all ultimately emit light in a continuous spectrum encompassing all colors, although at different relative intensities depending on their temperature.
There is another equally interesting mechanism that emits light with a certain amount of energy. When electrons make transitions from one orbit to another, they can gain or lose energy in certain quantities. This causes light to be absorbed and emitted in certain colors.
Because each element and each compound has a different number of protons and neutrons in its nuclei, the orbital levels of its electrons have specific energies. It is as if the totality of the orbits of each element were a ladder with steps of different sizes. So, in order to go up or down each staircase, we will need to make steps of a certain length.
This results in electronic transitions forming a discrete and characteristic spectrum. Each set of emission lines is like a bar code that shows the elements present in the emitting substance.
The best doctoral dissertation in the history of astrophysics
Cecilia Payne, armed with knowledge of spectral lines, took on the task of determining stellar composition. In 1925, he presented his results in his doctoral dissertation, considered to this day the best dissertation in the history of astrophysics.
Payne determined that hydrogen is the dominant element in the atmosphere of stars. He listed the elements discovered and established a connection between the spectra of stars and their surface temperatures. Thanks to spectroscopy, Payne made possible what Comte considered unattainable a hundred years ago: knowledge of the chemical composition of stars.
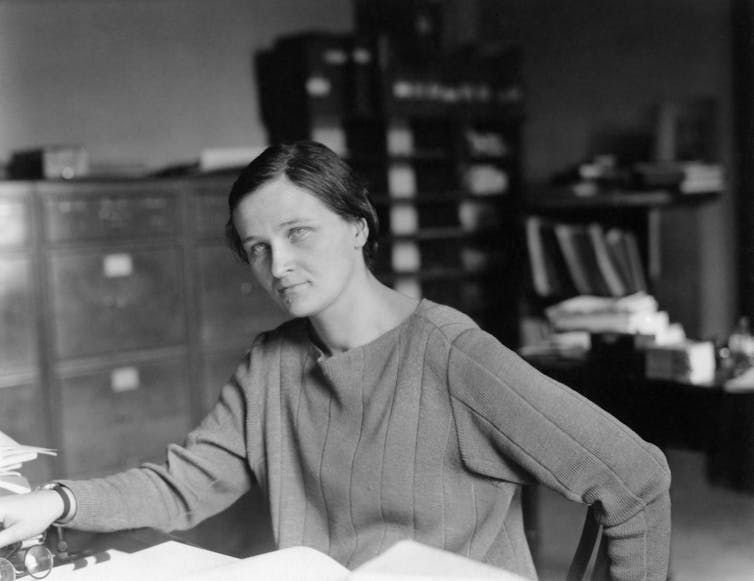
Smithsonian Institution/Science Service
From astronomy to the problems of this world
Today, techniques developed to study the stars are helping us solve Earth’s most pressing problems.
Spectroscopy can detect contaminants in air, water and soil.
We can detect toxic substances in the human body through anti-doping testing, perform quality and food safety testing, and perform tissue testing for non-invasive diagnostics.
To summarize, studying stars at large distances has provided us with the necessary tools to solve serious Earth problems such as environmental pollution, food pollution and threats to public health.