Second Brain – Elda Valley
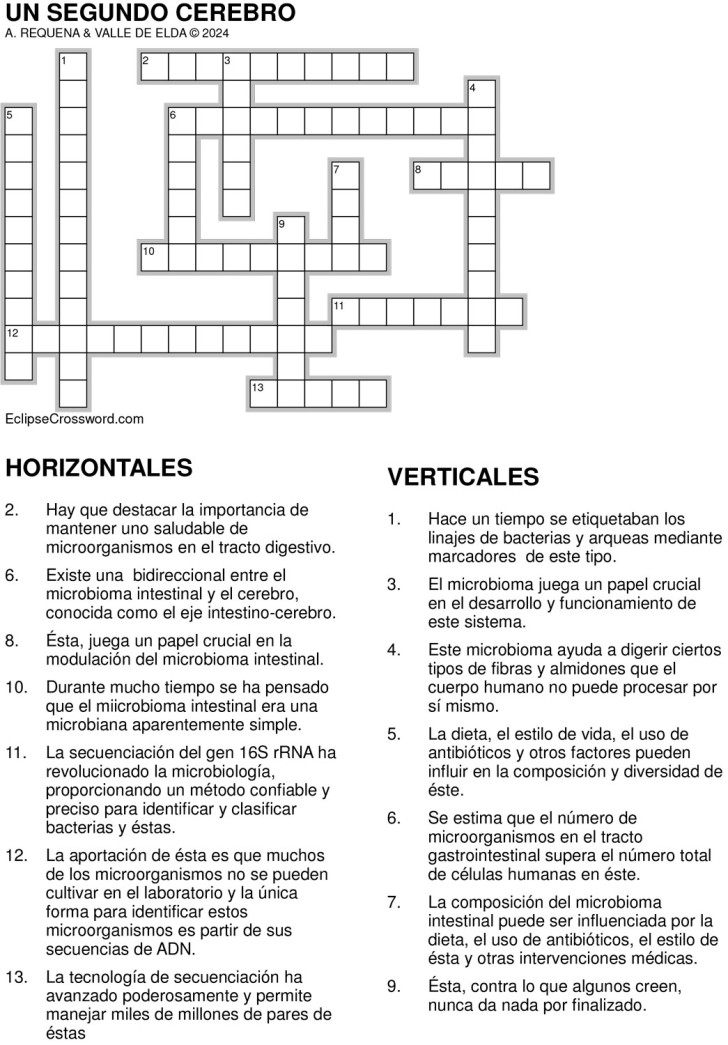
Science, contrary to what some people think, never brings anything to completion. Available analytical capabilities and technologies provide access up to a certain level. The depth achieved changes over time as the “accuracy” of the analysis changes over time. In particular, it affects the non-mechanical aspects of Nature. In biochemistry, discoveries usually occur as a result of the scope and scope of research. It is not so much a question of ignorance or previous misinterpretation as it is about the technological possibilities of accessing the intimacy of processes.
A prime example of this is the gut microbiome. It is unique for each person. There are types and groups of microorganisms that are common to many people. Diet, lifestyle, antibiotic use, and other factors can influence the composition and diversity of the microbiome. In addition to its role in the digestion and absorption of nutrients, the gut microbiome produces vitamins such as B vitamins and vitamin K. It also helps regulate the immune system and maintain the integrity of the intestinal barrier, protecting against pathogens. An imbalance in the balance of the gut microbiome, known as dysbiosis, is associated with a wide range of diseases, including inflammatory bowel disease, obesity, type 2 diabetes, cardiovascular disease and some mental health conditions such as anxiety and depression. Diet plays a critical role in modulating the gut microbiome. Fiber-rich foods such as fruits, vegetables and whole grains promote a healthy microbiome. Probiotics and prebiotics can also influence the composition and function of the microbiome. Gut microbiome research is a rapidly evolving field. Scientists continue to discover how this complex ecosystem influences health and disease and how it can be manipulated to improve health. The importance of maintaining a healthy balance of microorganisms in the digestive tract must be emphasized.
It is estimated that the number of microorganisms in the gastrointestinal tract exceeds the total number of human cells in the body. Most of these microorganisms live in the large intestine. These microorganisms live in a symbiotic relationship with the human body. Although they provide significant benefits to human health, in return the body offers a nutrient-rich environment for its growth. The gut microbiome helps digest certain types of fibers and starches that the human body cannot process on its own. These microorganisms break down these compounds into short-chain fatty acids, which are important for colon health and have beneficial effects on metabolism. It produces essential vitamins such as vitamin K and some elements of the B complex, which are critical for blood clotting, neurological function and energy metabolism. The microbiome plays a critical role in the development and functioning of the immune system. It helps train immune cells to distinguish between pathogens and benign microorganisms and can influence the immune response. It competitively inhibits the growth of pathogens in the intestine by competing for nutrients and adhesion sites, and by producing antimicrobial substances. There is a bidirectional connection between the gut microbiome and the brain, known as the gut-brain axis. Such communication can affect mental health, emotions and behavior.
The composition of the gut microbiome can be influenced by diet, antibiotic use, lifestyle, and other medical interventions. A diet rich in fiber, fruits, vegetables and fermented foods is beneficial for maintaining a healthy microbiome. Probiotics and prebiotics are also strategies used to positively change the microbiome.
It has long been believed that the gut microbiome is a seemingly simple microbial community. This is truly a group with an amazing diversity of cell morphologies. Analysis methods have evolved greatly. Some time ago, bacterial and archaeal lineages were tagged with fluorescent markers. Thus its structural and organizational diversity was studied until it was concluded that the complexity was much higher than that induced from morphology. Only in the first decade of the 21st century did the impetus come from the genomic revolution.
16S ribosomal RNA (16S rRNA) is a component of the 30S subunit of the bacterial ribosome, essential for ribosome function and protein synthesis. The gene encoding this RNA, known as the 16S rRNA gene, has attracted the attention of the scientific community due to its unique structure, which combines highly conserved regions with other, more variable ones. This feature makes it extremely useful for phylogenetic and taxonomic studies, allowing researchers to trace evolutionary relationships between different organisms.
16S rRNA gene sequencing has revolutionized microbiology by providing a reliable and accurate method for identifying and classifying bacteria and archaea. Conserved regions allow gene amplification by PCR (polymerase chain reaction) using universal primers, while variable regions provide the resolution necessary to differentiate closely related species. This has enabled the construction of extensive phylogenetic trees that “map” the relationships between microorganisms, contributing to a better understanding of their evolution and diversity.
16S rRNA gene analysis has had a significant impact on several areas of research and biotechnology. In microbial ecology, this has enabled the characterization of microbial communities in a variety of environments, from soil and water to the human microbiome, revealing enormous microbial diversity and its role in ecosystems. In medicine, 16S rRNA sequencing is used to identify pathogens in clinical samples, improving the diagnosis of bacterial infections and the selection of treatment options.
Despite its usefulness, 16S rRNA gene sequencing also faces challenges. Variation in variable regions can make differentiation between closely related species difficult, and the presence of multiple copies of a gene in a single genome can lead to ambiguity. In addition, this method does not provide information about the metabolic potential or pathogenicity of microorganisms.
The 16S rRNA gene has become a powerful tool in modern microbiology, essential for the classification and study of microbial diversity. Despite the difficulties, the application of this gene in research, biotechnology and medicine demonstrates its invaluable value.
The 16S rRNA gene has become like a barcode generator based on a short, unique gene sequence. This provided some taxonomic information and allowed us to determine when one microbe was phylogenetically related to another.
The situation has changed since the genomic revolution, and then it was a matter of analyzing a sample of the environment, extracting the DNA of all the microorganisms and then reconstructing them to analyze which microorganisms were identified in the sample, and even more, finding out the role they played. This is how the so-called metagenomics was born. This allows us to reconstruct the genomes of microorganisms in the environment.
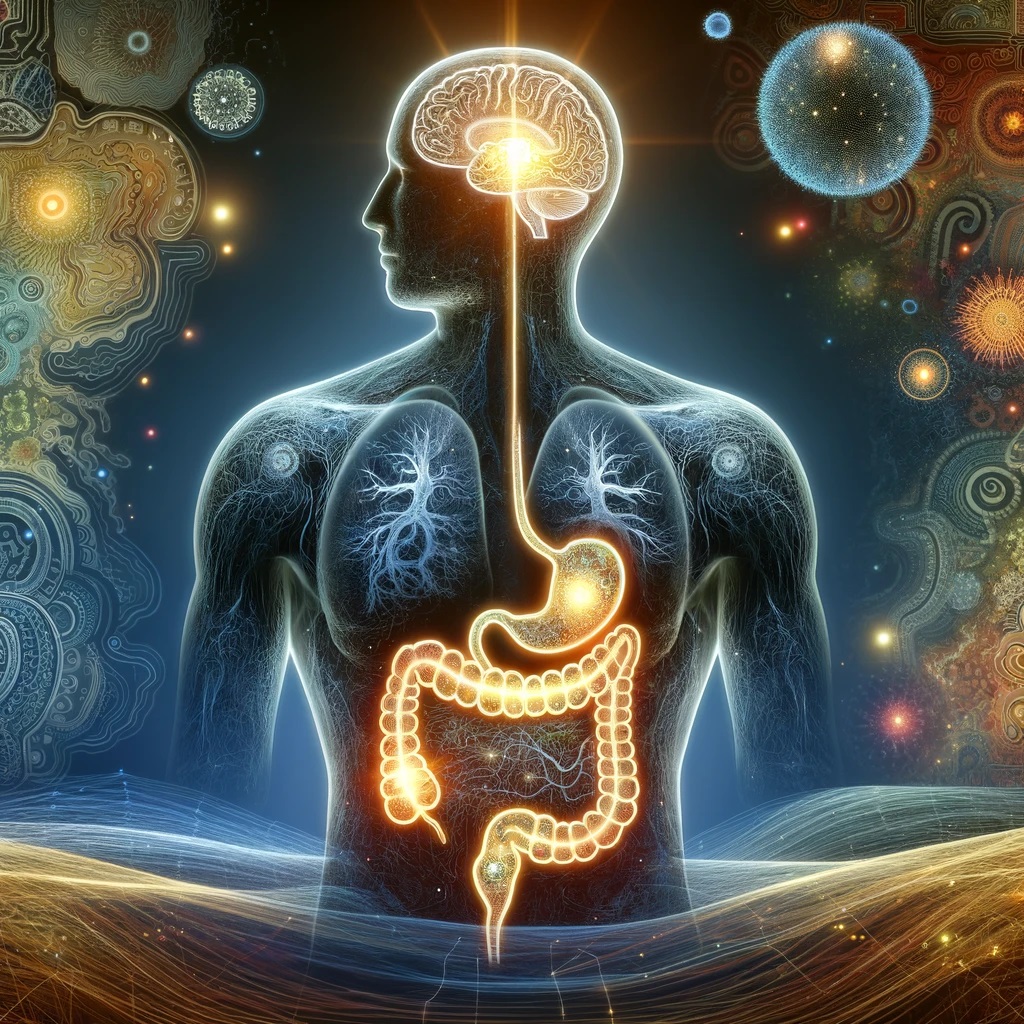
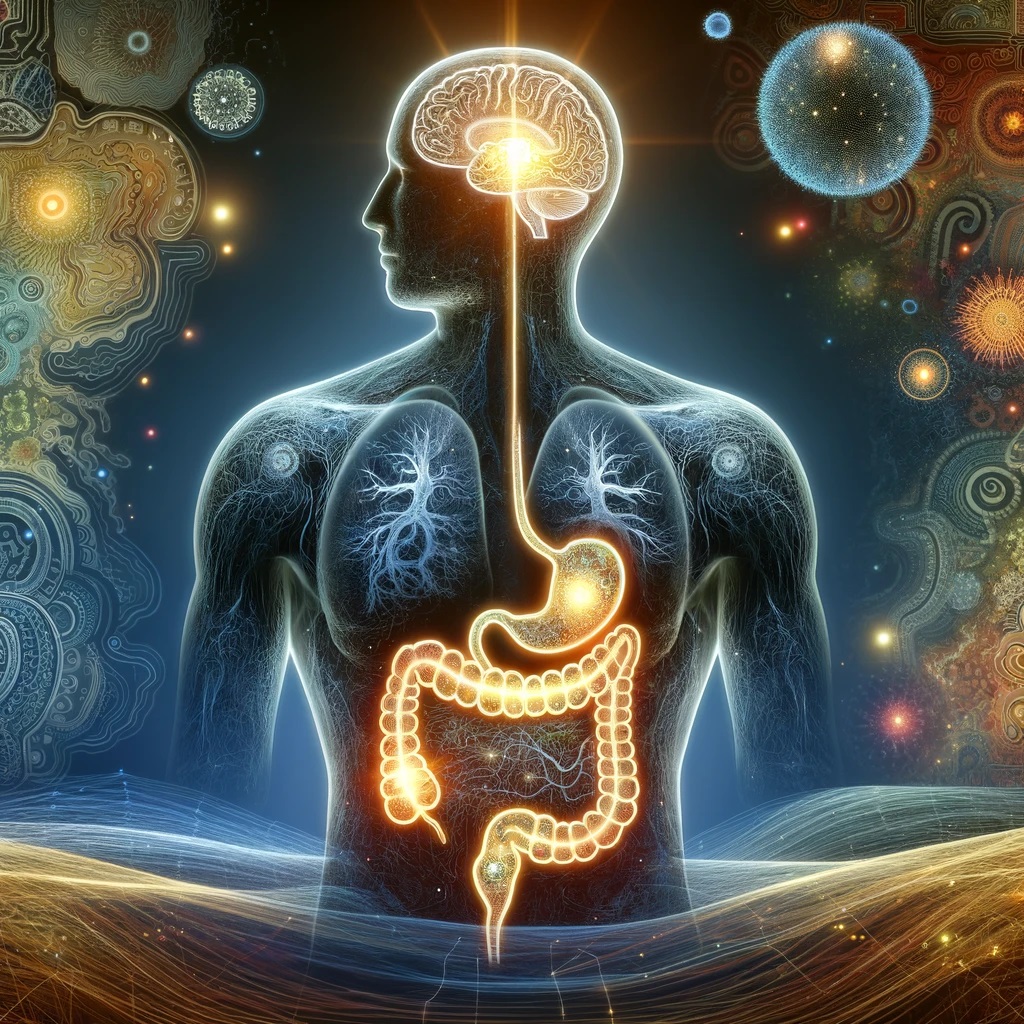
The contribution of metagenomics is that many microorganisms cannot be grown in the laboratory, and the only way to identify these microorganisms is to determine their DNA sequences. The path forward is clear: it starts with a large amount of DNA, which is randomly cut into fragments, which are sequenced and subsequently the genomes of all microorganisms are reunited. Not easy. If there is only one microorganism and only one genome, there is only one problem. But if there are many of them and their parts are mixed, there is only one way to reconstruct the genomes of each individual microorganism – using calculations, what is called bioinformatics.
Sequencing technology has come a long way and can process billions of base pairs. Techniques have also improved, with the introduction of computer-aided management of vast amounts of data, unimaginable just recently, and so-called metatranscriptomics, which analyzes the mRNA expression of entire microbial communities; the proteins they produce through metaproteomics, and then the resulting metabolites through metabolomics.
This is how we currently approach the study of the human microbiome. This interest is significant given that the gut microbiome is associated with a wide range of diseases, from immune to neurological, metabolic or cardiovascular diseases.
The ultimate goal of any study is to establish a cause-and-effect relationship. Not only define what it is, but also how it works. The practice of medicine is essentially a practice in which the authority references are usually largely empirical in nature. Scientists are trying to establish and substantiate a cause-and-effect relationship, as well as to establish direct connections and mechanisms that cause associations. This is the key to advancement and progress. Living biotherapies for treating disease rely on the ability to establish cause-and-effect relationships.
The importance of this type of research can be seen today when we have universal computing capabilities. Connections between the gut and the brain have been established, as well as links to anxiety, stress or depression. The bidirectional connection between the gut and brain, known as the gut-brain axis, occurs through neural, hormonal, and immunological pathways. This complex interaction means that the health of our gut can influence our mood, our decisions and vice versa. For example, stress can cause gastrointestinal symptoms, and gut problems can affect our mental health, contributing to conditions such as anxiety and depression.
Understanding that the gut can function as a second brain has expanded our view of human health, emphasizing the importance of maintaining good gut health not only for physical health, but also for mental health. As research in this area advances, it promises even greater discoveries about how our body and mind are connected, which could lead to new therapeutic approaches for a wide range of diseases.