They are creating a miniature particle accelerator for medical use.
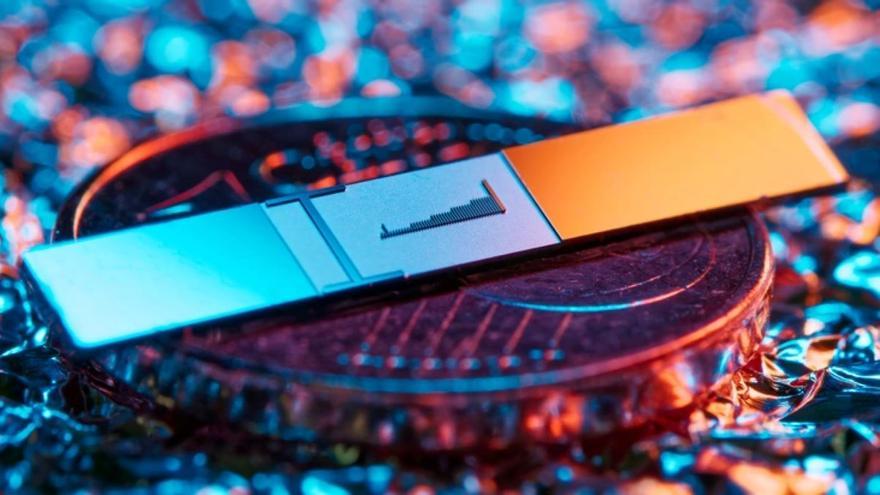
German researchers have developed an electron accelerator just under half a millimeter long and 0.2 micrometers wide, or less than a thousandth of a millimeter. It has applications in basic research and will allow the creation of new radiation therapy tools. Interview with the main characters Peter Hommelhoff and Stephanie Kraus.
Connected
From the world’s largest circular particle accelerator, the Large Hadron Collider, to CT scanners in hospitals, particle accelerators have become indispensable in many areas of research, medicine, and industry.
The machines come in a variety of sizes and can be used to probe fundamental questions in nature, research new materials, or treat people with radiation therapy.
Although Rolf Widero developed the basic principle almost a century ago, researchers around the world continue to work on new concepts for particle acceleration. Among other things, with the goal of creating smaller and cheaper accelerators.
Peter Hommelhoff And Stephanie Krausfrom the University of Erlangen-Nuremberg, explain in an interview with World of Physics how they developed a new particle accelerator in a miniature format and what applications it promises.
What is a particle accelerator?
Peter Hommelhoff: In a particle accelerator, smaller particles such as electrons or protons reach very high speeds. The higher the speed, the more energy the particles have. In most cases, oscillating electric fields are used to accelerate them. Electrically charged particles experience the force of an electric field. Currently, high frequency fields in the microwave range are mainly used. Particles are accelerated in special channels in a vacuum. The shape of the channel determines the internal field. Therefore, the accelerator is configured so that the particle packets experience a forward force as a single unit. The particle accelerator also has the function of holding particle packets together. The ultimate goal is to create a sufficiently large flow of accelerated particles.
What types of particle accelerators are there?
There are two types of particle accelerators: linear and circular. In a linear accelerator, particles are accelerated in a straight line, and in ring accelerators, particles are carried along a circular path in a ring-shaped system by additional magnetic fields. The advantage of these circular systems is that the accelerating structures can be used multiple times for the same batch of particles. This allows particles to be accelerated to much higher energies. The Large Hadron Collider (LHC) at the CERN research center is one such circular accelerator. There, protons or heavy atomic nuclei are accelerated to almost the speed of light.
Why do people care about accelerated particles?
Accelerated particles are important for many areas of research and applications. For example, at the LHC, physicists need highly accelerated particles colliding with each other to study the fundamental components of matter. X-rays can also be generated by fast particles colliding with a piece of metal. This radiation is subsequently used in medicine for diagnostics and radiation therapy, as well as in materials science. Besides, the call is very interesting synchrotron radiation, which particles emit when magnetic fields force them to follow an oscillating path. For example, physicists, chemists and biologists use synchrotron radiation sources such as PETRA III at the DESY research center in Hamburg to study the structure of molecules or solids. This radiation can also be used to study very fast processes in molecules so that films of chemical reactions can be created.
Why do particle accelerators have such different sizes?
This is because there is a maximum energy gain per distance that particles can experience when accelerated, called acceleration gradient. It is limited by the destruction threshold at which the fields become so large that the structure of the accelerator (in the case of microwave accelerators, its surface) is damaged. Therefore, the length of the acceleration path depends on the energy of the generated particles and the maximum achievable acceleration gradient. In general, the higher the desired energy, the greater the distance. For example, the European X-ray laser (European XFEL in Hamburg) or LCLS in California are several kilometers long. But linear accelerators, which are typically just one meter long, are also used in medicine. In our opinion, it is enough for the accelerator to be only one millimeter long.
You have built a miniature accelerator. How it works?
We have developed linear accelerator very small for electrons just under half a millimeter long. Our acceleration channel consists of columnar nanostructures arranged in two rows. This channel through which electrons move is only 0.2 micrometers wide, that is, less than a thousandth of a millimeter. Let’s illuminate the structure from above with pulses of laser light and create an oscillating electric field in the channel. The important thing here is that laser particle acceleration only works on electrons that are already in motion. Thus, in front of our accelerator there is an electron source that produces fast electrons. Together with the necessary electronics, nanostructure and laser system, this is our particle accelerator.
How is the accelerator designed?
Stephanie Kraus: We fabricate our accelerator structure from silicon, which can be thought of as a dielectric for near-infrared laser light. These dielectrics, including glass, for example, conduct little or no electricity. Since the material does not absorb light, the damage threshold is significantly higher compared to the metal of conventional accelerators. This allows us to create very strong electric fields with the laser system and thus accelerate the electrons more strongly. To create a nanostructure, we use so-called photolithography. The process is also used in the production of smartphones and computer chips. We use a dry process to specially etch tiny structures in the dielectric.
What new things have you achieved now?
Peter Hommelhoff: In 2013, in parallel with researchers at Stanford University in California, we demonstrated for the first time that electrons can be accelerated through very simple structures using laser light. However, the particles were not yet bound by optical forces. A few years later, colleagues from Darmstadt theoretically demonstrated that particles could be held together using lasers and a suitable nanostructure.
Stephanie Kraus: Then we had to work hard and in the end it paid off. In 2021, we investigated how electron beams can be held together and developed a corresponding channel through which the electrons are then sent. The next step was to further develop the nanostructure so that the particles were optimally accelerated and kept together at the same time. To do this, we carry out complex modeling. The next hurdle was getting the structures right. And we finally saw how well our new accelerator works. We noticed that the electron energy increased by 43 percent. This made it clear: our accelerator works!
What could the mini accelerator be used for in the future?
Peter Hommelhoff: This technology has many applications in basic research and medicine. We hope to use this to create new radiation therapy tools, because by allowing our accelerated particles to collide with a piece of metal, we could generate the X-rays needed for radiation therapy. For example, we are partnering with medical technology companies for the first application on a compact device, ideally the size of a mobile phone. People with skin cancer can potentially be treated with radiation therapy. If the entire system were integrated on one chip, can be mounted on the endoscope as a pen tip. It could even be possible to irradiate tumors directly in the body in a minimally invasive manner.
What are the next steps in your research?
Stephanie Kraus: First of all, it is important to continue research into the material of nanostructures in order to increase the destruction threshold and thus achieve greater acceleration. Instead of silicon, we could use glass, that is, silicon dioxide. But for this to happen, it is necessary to adjust a number of stages of the production process. We would also like to further optimize the conduction of electrons through the channel. We are also still limited by our laser system and would like to work on it in the future.
Peter Hommelhoff: A serious problem at the moment is that the number of accelerated particles is too small. Because our accelerator designs are very small, they can only fit a few electrons. That is why we are working to increase the particle flow. For example, with a better source of electrons, the current could be increased directly. But if you change the source, you will also have to adjust the throttle, and vice versa. For this reason, we can only take small steps in our research. But the good thing about physics is that these small and sometimes slow steps can ultimately go very far.
This is an interview originally published in the German journal Welt der Physik, published by the Federal Ministry of Education and Research and the German Physical Society. Reproduced with permission.